Could this man hold the secret to human regeneration?
Could This Man Hold the Secret to Human Regeneration?
Michael Levin wants to help people regrow lost limbs. Now he could be on the verge of a breakthrough.
This piece was awarded the 2013 IOP-STFC Journalism prize.
Then there’s regeneration.
A future in which humans regrow lost or diseased body parts feels like a mirage. But why? After all, many species can accomplish the task with ease. A decapitated flatworm, for example, will grow a new head, replete with a new brain. For the first week of their lives, tadpoles can replace lost tails. And the axolotl, or Mexican salamander, has the ability to regenerate everything from its limbs and tail to its spinal cord and skin, all without any evidence of scarring. Even some mammals have limited regenerative abilities: every year, reindeer regrow their shedded antlers. And, in some circumstances, young rats that lose a leg can grow it back.
Humans have a sliver of regenerative capacity, too. If a child experiences a neat slice through the end of his fingertip, that tip will grow back — although the ability disappears sometime around the age of 12. The Greek legend of Prometheus, the god who was cursed to have an eagle peck out his liver each day, only to grow it back overnight, actually contains a grain of physiological truth: if you were to lose part of your liver, it would, in fact, repair itself. With the exception of our skin, it’s the only human organ that can do this.
Regenerating a small body part under special circumstances is one thing, but what if we could regrow entire lost limbs? What if we could signal to our bodies to regrow damaged retinal tissue — or even to regrow an entire eye? Michael Levin doesn’t think this is an outlandish fantasy. In fact, he thinks he may be on the path to figuring out how to do precisely that.

Levin is director of Tufts University’s Center for Regenerative and Developmental Biology in Medford, near Boston. He’s a 43-year-old Russian émigré who looks like a geeked-out Gen Xer: His smooth hair is parted far to the side; a neat geometric beard frames his face; and he’s most comfortable in a college uniform of T-shirts over long sleeves.
His life, however, is marked by a willingness to embark on undertakings that many would consider arduous in the extreme. He possesses exceptional focus and personal conviction. These traits are common among successful scientists, and essential for any who want to pursue a leftfield line of enquiry.
Levin thinks that the key to regeneration — the key to pattern, to shape — may be found in the electrical signals that are transmitted among all our cells, much like the ones and zeros that zip around in a computer’s hard drive. Manipulating these signals has already allowed Levin to produce results more suited to an X-Men comic than a scientific journal, including the creation of four-headed flatworms. Over the course of the next year, he will begin experiments that could make human regeneration a reality.
Levin’s work is little known, perhaps because so many scientists believe that the key to human regeneration — if such a thing exists — lies in studies of genetics and stem cells. Such studies have produced incredible results: a patient’s windpipe, repaired in a lab; a segment of functional bladder, fashioned on an artificial lattice. These achievements offer the hope that a patient will one day be able to grow a new organ from her own cells, instead of waiting for someone else’s misfortune to be her good luck.
But none of these accomplishments involve recreating the complex organs and limbs that our bodies produce naturally. Scientists can’t command the cells at an amputated elbow to generate a limb replete with muscles, tendons, bone, cartilage, blood vessels. No one has found the signal that gives the command: “Become an arm.”
At least not yet.

ONE NIGHT IN THE LATE 1700s, Luigi Galvani, an anatomy professor at the University of Bologna, Italy, stood on his balcony and lined up a string of butchered frog legs. This in itself was not unusual — they were, in all likelihood, awaiting the dinner plate. But on this night the air was crackling with electricity from a storm, and Galvani noticed something odd: when he touched the legs with a pair of scissors, they twitched.
The professor’s curiosity was piqued. Soon after, he laid out some dissected frog legs in his laboratory — where, as it happened, he also kept a newfangled machine that stored static electricity. Any time the machine was on and someone touched the legs with a metal scalpel, they jumped. Galvani wondered if the limbs contained some sort of charge, an “animal electricity” essential for life. He thought it might come in the form of an undiscovered biological juice and, while he was wrong about that, he had become perhaps the first person to purposefully stimulate exposed nerve cells with electricity.
In the years that followed, Giovanni Aldini, Galvani’s nephew and former assistant, took the experiments further. In 1802, Aldini connected a primitive battery to a recently severed ox head. It was as if the animal had come back to life: its eyes flew open; its ears wriggled; its tongue jerked. He attempted a similar experiment on the corpse of a murderer who’d been hanged in London’s infamous Newgate Prison. The effects were much the same, Aldini later wrote: “The jaw began to quiver, the adjoining muscles were horrible contorted, and the left eye actually opened.”
These ghoulish experiments were well known in scientific and popular circles — Mary Shelley used the notion that electricity could animate matter as the foundation for Frankenstein — and interest in the effects of electricity on living creatures continued for the next 150 years. Many efforts, including using electricity to treat hysteria and melancholia, amounted to little more than quackery. Fringe thinking may have helped sideline the study of bioelectricity.
In the end though, it was overtaken by a rival branch of science that seemed to hold the key to all biology’s secrets. When the structure of DNA was discovered in the 1950s, the search for the commands that shape our bodies became an investigation into the extraordinary interplay between genes and proteins. The studies that descended from Galvani’s were all but swept away.
It’s odd that electricity has been so neglected, because it is everywhere in our bodies. Ions flow in and out of our cells. Voltage pulses speed down our nerves. We are, in effect, walking electrical networks. The importance of electricity is well understood when it comes to the nervous system and the heart — think of the electrical defibrillator pads used to revive someone after a heart attack. But in many ways, we remain stuck in an eighteenth century mind frame, aware of the electric signals that course through us but oblivious to the ways in which they could play a subtler, and more profound, role in our development.
Reviving bioelectricity would require someone who knew little about the frivolous side of bioelectrical research, and who wasn’t concerned about how his interests would appear to colleagues.

MICHAEL LEVIN WAS BORN in Moscow in 1969, around the height of the Cold War. His father, Benjamin, was a successful computer engineer; his mother, Luba, was an accomplished concert pianist. Like many Jewish families in the Soviet Union, life was not easy for the Levins. They lived in a cramped one-room apartment, and Michael’s parents carried the memories of family members lost to Stalin’s purges of the 1930s. Benjamin and Luba tried to shelter their asthmatic son as much as they could, but there were realities they couldn’t hide from their only child, including the regular beatings that Jews suffered for no reason other than their religion.
Michael spent his early years at home with his parents, who encouraged his fascination with the world around him. He remembers his father taking the back off the family’s television when he was four or five and thinking, “Someone was able to figure out how all those different parts fit together — it wasn’t an accident!”
Benjamin, meanwhile, remembers Michael at around six years old, obsessing over the crawling, squirming creatures he discovered while digging in the dirt. “He would watch them move, and he was always asking me questions — ‘Why?’ ‘How?’ — that I wasn’t really qualified to answer,” says Benjamin.
Soon father and son began to read science textbooks together and, by the time Michael was eight, he had immersed himself in university-level physics and biology. The math was incomprehensible, but the descriptions of atoms and solar systems and the natural world were intoxicating. More than three decades later, Levin still remembers learning that just one word— ‘frequency’—can describe everything from radio to light to gamma rays. It was, he says, one of “the most beautiful concepts ever,” and it led him to speculate that “living things must be able to take advantage of the laws of physics, not just chemistry”. This seemingly prosaic conclusion would have huge consequences for Levin’s career.
For the first six or seven years of Michael’s life, the Levins didn’t dare dream of escaping the Soviet Union. Then, in 1976, Benjamin learned that a select handful of people had been allowed to leave. He put in a request to emigrate. It was a risky move. Benjamin remembers a black joke common at the time: those who applied had a 50-50 chance of being sent West — to freedom — or East — to Siberia. The Levins were fortunate, and were among the first wave of Russian Jews that left for the United States in the late 1970s. They sold Luba’s piano and Benjamin’s father’s stamp collection to pay the exit fees, which equaled about eight months of Benjamin’s engineer’s salary. Michael celebrated his ninth birthday in Italy, a stop on the journey to the Boston suburb of Lynn, where they joined a growing community of Jewish émigrés from the Soviet Union.
The move was not an easy one for Michael. He didn’t speak or understand English, and it was a year before he could express himself fully. Even today, a faint memory of the Soviet Union lingers in his speech, as his Russian-inflected tones occasionally clip against flat Boston vowels. But even though language was tricky, the opportunities to explore seemed limitless. In 1979, Benjamin got a job at Digital Equipment Corporation, a computer manufacturer, and began bringing home unwanted electronics. With help from his father, Levin quickly learned how to code, and soon asked his parents for a RadioShack TRS-80, one of the first home computers available in the United States. Benjamin remembers paying about $1,000 for the machine, a significant outlay for them.
“I don’t know too many works of science fiction that are as wild and out there as things that have been discovered by real science.”
The Levins were not quite as indulgent when Michael, like the rest of the country, got swept up in the Pac-Man craze. Levin begged his father for the game, to no avail. If he wanted to play Pac-Man, Benjamin told his son, he’d have to build it himself. Michael decided to do just that.
Night after night, he perched in front of his TRS-80, which he hooked up to an old black-and-white TV. The computer had no long-term memory. The day’s work would vanish every time he shut it down, so Michael meticulously transcribed everything he did in an 8.5 x 11-inch spiral notebook. When he next sat down to work, he first had to re-enter everything he’d done previously. He was 11 years old.
Within six months, Michael had created a working facsimile. His Pac-Man moved through a maze, chomping dots and escaping ghosts. Unlike the commercial edition, the maze in Levin’s version was different in every game; what’s more, the ghosts never clustered together, making them harder to avoid. It was a challenging game but, after all his efforts, Michael spent little time playing it. He realized it was the creative process that had been driving him, not the end product.
Michael’s interests extended beyond computers. In 1981, soon after the Levins moved to Swampscott, a neighborhood just north of Boston, he turned a small room on the second floor into a makeshift biology laboratory. Levin used it to explore such questions as the line between life and death, which he investigated by chilling snails and insects in his parents’ freezer.
But to satisfy his ambitions he had to look beyond what was available locally. Aside from some electrical parts available at Radio Shack, he couldn’t find any of the equipment he wanted. He found the solution at his local library, in a reference book that contained details of a company that provided science materials to high schools and colleges. Levin knew that a random teenager living in suburban Boston wouldn’t be allowed to buy lab equipment and formaldehyde-preserved rat’s and sheep’s brains, so when he called he identified himself as a representative of the imaginary “Saint Augustine School of Science.” The employee who took his order only wanted one more piece of information: a reference number for the order. “I had no idea what that was, so I’d say, ‘Um, seven?’ says Levin. It worked.
Levin’s closeness with his parents, and the lengths they went to indulge his curiosities, continued throughout high school. The summer after his sophomore year, Michael, Benjamin and Luba travelled across North America to Vancouver for Expo ’86, a World’s Fair. Father and son wandered into a local bookstore, where Michael chanced upon The Body Electric, co-written by an orthopedic surgeon named Robert Becker.
Becker had held a senior position at the Veterans Administration Hospital in Syracuse, New York, but he was also something of a crank. He’d been an early and vocal proponent of the theory that power lines cause cancer and, in The Body Electric, argued for a link between electromagnetic fields and a grab-bag of other conditions, including Reye’s syndrome, Lyme disease, Legionnaire’s disease, AIDS and genital herpes. His own research went largely unfunded, Becker implied, because of the meddling of “politicians, military leaders, and their lap dog researchers.” As the Los Angeles Times put it in a review, “so many of his proposals for study met with rejection, and he feels the knowledge to be gained from his work is so critical for human survival, that Becker is submitting his thesis to the public.” The Times went on to note that Becker “sometimes sounds paranoid”.
Despite these eccentricities, the book is one of the few comprehensive texts on the role of electricity in regeneration. In the first 200 pages, Becker describes forgotten experiments from the early twentieth century that suggest electricity can spark the regrowth of body parts. In one notable study, scientists used electrical currents to prompt a small freshwater animal called a hydra to regrow a head in place of a tail.
The scientific establishment had a hard time separating this research from Becker’s claims about links between electromagnetic fields and extra-sensory perception, as well as his suggestion that admittances to mental hospitals were linked to solar flares and magnetic storms. Seventeen-year-old Michael Levin did not. He was transfixed by the description of bona fide scientists exploring the very questions he’d been asking himself. (In his home lab, he’d been applying currents to protozoa to see if this would make the single-celled organisms grow more quickly.) According to Becker, electrical currents played an even more profound role in animal development than Levin had ever imagined.

TWO YEARS LATER, Levin enrolled in computer science at Tufts University, just a half-hour drive from his parents’ house. Biology was supposed to be a hobby, but with his interest kindled by what he was reading, it began to take up increasing amounts of his time. Throughout his years at Tufts, Levin continued a project he had begun in high school: chasing down all the studies mentioned in The Body Electric, and every paper referenced in every single one of those studies — a mammoth undertaking in those pre-internet days. The result was arguably the world’s most extensive bibliography of research into bioelectricity, and its contents gave Levin a masterclass on the methods and techniques of the handful of scientists who had continued to study bioelectricity into the 1970s and ‘80s.
Looking back, Levin says the book so consumed him because its entire premise supported what had become one of his fundamental beliefs: electrical signals are such a powerful way of delivering information that evolution must have found a way to take advantage of them. He also loved the obscurity of the field, and the medical benefits that might flow from it. “When I picked up that book and went through it,” he says, “I felt that this was an area worth devoting my scientific career to.”
In 1992, Levin graduated from Tufts with a dual degree in computer science and biology, and enrolled in a PhD in genetics at Harvard Medical School. There he’d eventually work with Cliff Tabin, who was known for his work on embryonic development. In Tabin’s lab, Levin identified the genetic pathway that determines how cells in a chick embryo know to put the heart on the left side of the body. Later, he discovered a chemical that enables the two sides of the body to communicate and determine which side will develop into which. (Nature would call this work a “key breakthrough” and a “milestone” in the past century of developmental biology.)
“The main lesson from biology is that the main stuff is all very similar. And there really is no principled reason to think it’s not going to work.”
As it happened, the movement of that chemical reminded Levin of the movement of a charge in a battery-driven circuit, which prompted him to wonder whether there was a bioelectric signal that set the whole process in motion. It turned out there was. Tabin says that, to this day, he doesn’t believe anyone other than Levin would have thought to look for this connection.
Those were satisfying years for Levin. In 1999, he began dating Kristin Willoughby, a computer programmer he met online. (The pair would wed two years later, and go on to have two sons.) That same year, Levin noticed that the Forsyth Institute, an independent organization affiliated with the Harvard School of Dental Medicine, had an opening for a developmental biologist. He applied almost on a whim — and was not only offered the job, but told he could pursue any line of investigation he wanted, so long as he could drum up funding. He would spend the next eight years at the Forsyth, where he would finally start testing his theories about regeneration — theories that had been percolating for more than a decade, ever since he’d picked up a strange book in a used bookstore in Vancouver.

BY THE TIME LEVIN WAS thirty-one years old, he’d formulated the questions that would shape his life’s work. What is the role of the body’s intrinsic electric signals? How do they interact with genes and proteins? How do they affect the development of an embryo? To find the answers, Levin picked up a thread that led back to the work of the bioelectricity pioneers 60 years earlier: he set about trying to control regeneration in animals that already had some regenerative abilities.
He began with the African clawed frog, or Xenopus laevis, a bloated-looking creature that had been used in some of the studies Becker wrote about. A Xenopus tadpole can grow back its tail, provided it’s lost during the first seven days of its life. But from day eight — right around the time the tadpole begins to metamorphose into a frog — it begins to lose that capacity, and at ten days the ability has gone completely. Levin thought that the regeneration signal — the thing that instructs a tadpole’s cells to form a replacement tail — was what stopped functioning during the transition period. If that signal was an electric one, as he suspected, and if he could figure out what it looked like, perhaps he could work out how to turn it back on in tadpoles that had entered the non-regenerating stage.

Levin’s intuition told him to start studying the electrical properties of cells. Cell walls are dotted with pumps and channels that pull charged atoms — calcium, potassium, sodium — into the cell and spit them back out. As charge builds and ebbs, the voltage across the cell changes. And changes to this voltage, thought Levin, might be the signal that triggered regeneration in the experiments that Becker had catalogued. If so, the secret to regrowing limbs would lie in controlling the flow of charge across cell walls.
But which cells? And which channels? Levin decided to work backwards, to figure out if he could stop regeneration in tadpoles by switching off a particular channel. It was not an easy task. First he had to compile a list of the hundreds of drugs that act on channels, a painstaking project that took months to complete — there are drugs that block a single channel, and drugs that take out an entire family of channels. There was also the risk of side-effects. When Levin started experimenting with drugs from his list, colleagues told him he was wasting his time: surely closing off an ion channel in a days-old tadpole — a channel that is present in cells throughout the body — would kill the tiny creature.
The tadpoles didn’t die, and within a few months Levin had narrowed in on concanamycin, a drug that disables a pump that propels hydrogen ions across cell walls. Dosed with the drug, tadpoles that should have been able to grow back their tails were no longer able to.

In the autumn of 2005, Levin took a tadpole that was too old to regenerate a tail, amputated its tail, and added a cell-wall pump that he hoped would signal to the tadpole that it was still young enough to grow a new tail. Any number of things could have gone wrong. The tadpole might have grown a tumor or an elephantine tail, or have only produced a tiny bud — or even a leg. Or it might not have grown anything at all, which was Levin’s biggest concern.
But it worked. The tadpole grew its entire tail back. Levin had triggered regeneration in an animal that should have lost that ability.
Levin does not lack self-confidence. Even so, he was astounded at his experiment’s success. “Boom!” he says, describing the perfect tail that was produced. “That was the most amazing thing. Without controlling the…pump, and without tweaking the specifics of it, you get a perfectly normal tail that knows when to stop.”
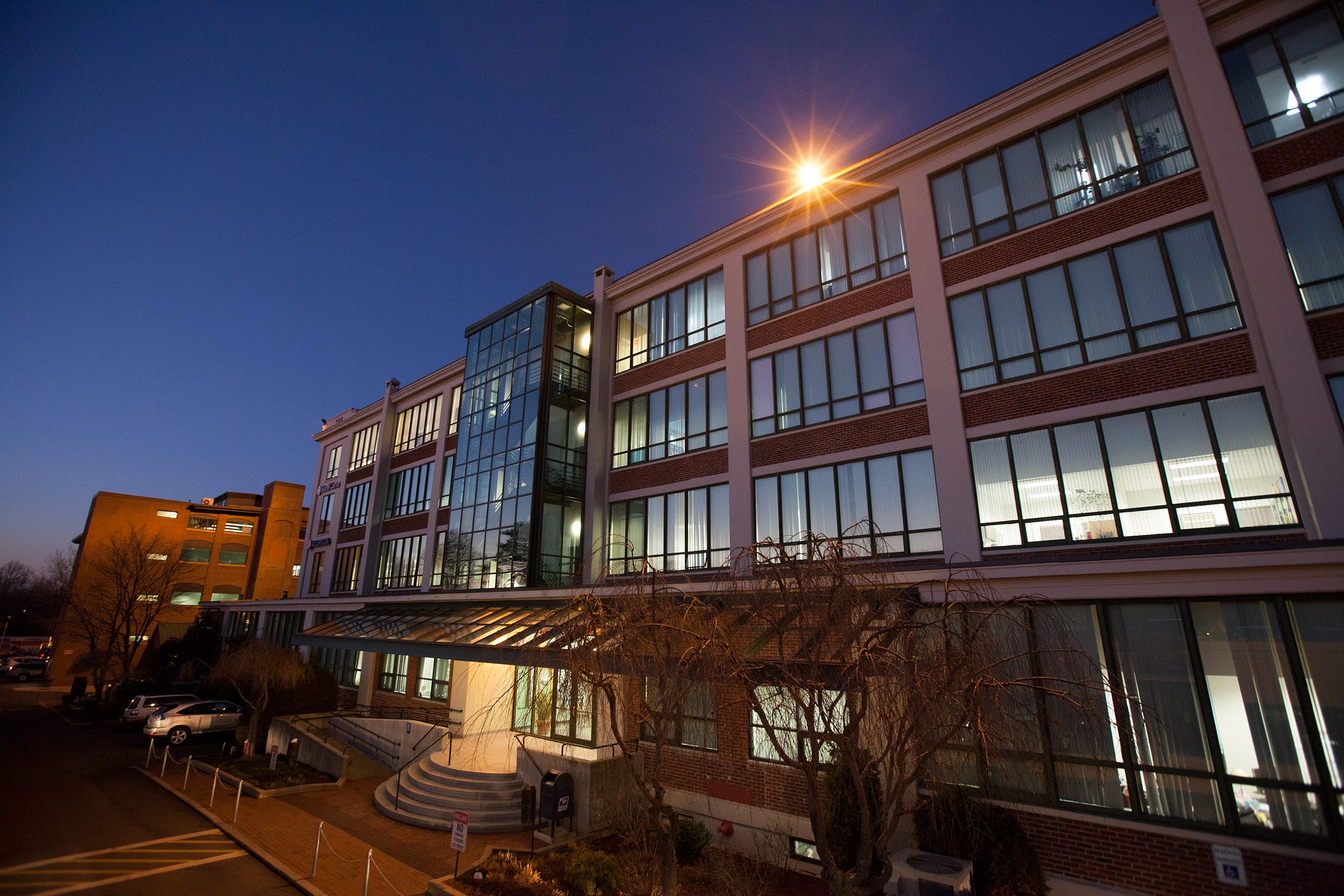
THE BOXY FOUR-STOREY BUILDING that houses the Tufts Center for Regenerative and Developmental Biology is tucked behind a parking lot off a nondescript two-lane road in Medford, Massachusetts. Levin’s lab has been housed on the top floor here ever since his alma mater lured him from Forsyth in 2008. Posters of blown-up covers of scientific journals line a hallway; one of them features a crimson-hued four-headed flatworm. Levin’s team has figured out how to manipulate these centimetre-long creatures so that they grow in a variety of ways — a head at both ends, or no heads at all. The “monsters,” as one of Levin’s post-docs affectionately calls them, live in plastic containers filled with Poland Spring water — the only water in which the worms thrive and regenerate properly, and the same water that’s available in the lab’s snack room — and are fed 100% organic beef liver. Examined under a microscope, the mottled beige and brown worms twist and twirl their cartoonish bodies into knots, with triangular heads peeking out every which way. They recall the world of Doctor Doolittle, these skinny pushmi-pullyus with a head at either end of their bodies.
Around a corner from the worms, transparent tanks contain fist-sized Xenopus frogs. There’s a thrum from the system that filters and irradiates tap water, before adding the salts and minerals necessary to mimic the animals’ native environment. The space smells faintly of marshland. In a neighboring room, technicians hand off Xenopus eggs to waiting scientists. The eggs have been inseminated and wiped clean of the gel that would normally cause the poppy-seed sized spheres to clump together on a rocky river bottom. In here, Levin’s team alters the ion channels — and thus the voltage of particular cells — in the frog embryos. On the wall, Levin has hung two framed copies of Galvani’s research drawings, including one of the frogs’ legs on Galvani’s balcony.
If Levin is able to unlock the riddle of human regeneration, the research will be done here, in the lab that bears his name. These days, much of that work is devoted to understanding various aspects of development. As an embryo begins its path to creaturehood, it undergoes dramatic changes. One cell divides into two, two into four, four into eight, continuing until there are dozens, thousands, millions of them. In the process, some cells transform into blood, others into bone; some into tissue, others into tendon. They organize into complex and interdependent structures: the hemispheres of the brain, an elbow that trails to wrist and fingernails, a heart tucked between a pair of lungs.
To get a tadpole to regrow a tail when it shouldn’t have been able to, Levin had to unlock one part of the regeneration code through a laborious process of trial and error, testing various drugs until he identified one he believed might work. But if animals do, in fact, store information about their shape in electrical signals, Levin will need to figure out how to actually read those signals — and that will require deciphering a whole new language.
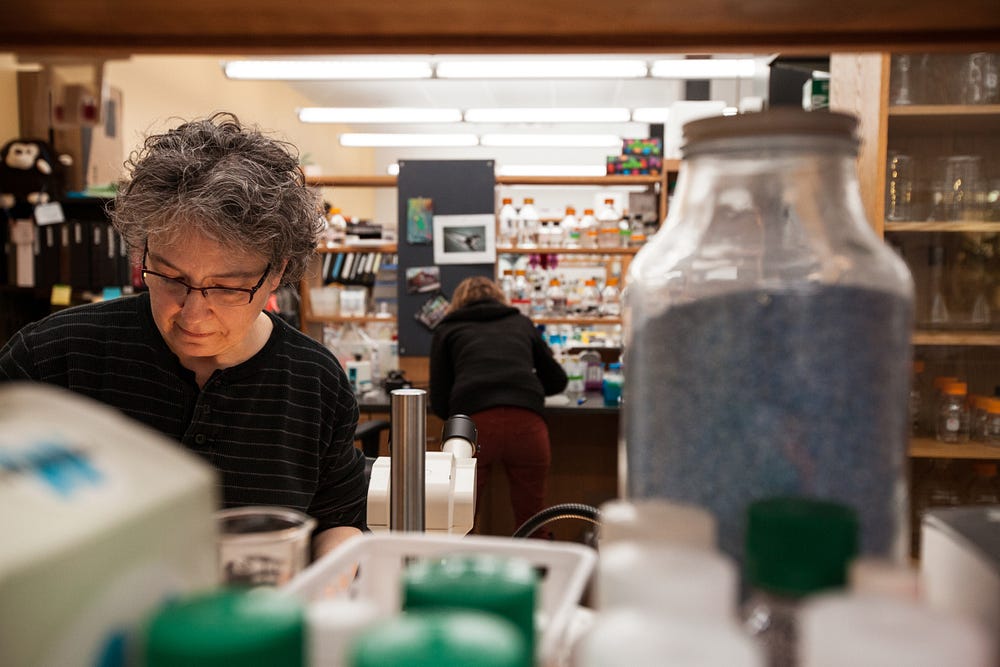
One of Levin’s most important collaborators in this quest is Dany Adams, an associate professor who, over the past ten years, has become the closest thing Levin has ever had to a research partner. There’s an odd-couple feel about their collaboration: Levin is the group leader who has been focused on his goals since he was a teenager; Adams is several years older and taught developmental and cell biology at Smith College, a premier liberal arts university, before realizing she wanted to return to research. Levin’s neat appearance contrasts with Adams’ tousled grayish-brown curls; he is quiet and reserved, she is voluble and punctuates her speech with waves of her hands; he tends to work in his office, while she prefers bustle and frequently takes her laptop to coffee shops.
What the two do share is an ebullient curiosity. It was Adams who, more than three years ago, found a way of visualizing the role that voltages play across the head of a developing embryo. One evening in September 2009, she was looking at a tadpole embryo under a microscope. The embryo had been treated with special dyes, which caused cells in the rapidly dividing mass to glow more brightly as they approached certain voltages. Adams often set up a camera to capture pictures from these tiny light shows, and on this particular day, the resulting images were unusually clear. She left the camera on overnight, set to capture a new picture every fifteen minutes. By the next day, Adams had 64 individual pictures — the equivalent of 16 hours worth of tadpole development, which she then compressed into a single, 13-second video.
The result is so remarkable it almost doesn’t seem real. As cells divide within the ball of the embryo, lines and shapes glow and disappear. A slash where the mouth will form shimmers into view, only to quickly fade away. A dot, signifying an eye, appears briefly on the left side of the embryo; a moment later, a matching dot flashes on the right.
Vertiginous time-lapse photography is a staple of nature documentaries, but this is different. These features — the mouth, the eyes — didn’t actually exist. In fact, many of the genes that are linked to their development hadn’t even been turned on. It’s only after the patterns fade, the ghost of features yet to come, that all the necessary proteins are activated. The video is a document of a previously unrecognized phenomenon: Adams had filmed the blueprint of a tadpole’s face before any currently available tools could indicate that it would even appear.
Adams believes that the flashes in her light show represent a series of switches being flicked on at different voltages. When a cell reaches a certain voltage, she says, the activity of the cell’s genes changes, triggering a chain of events that lead to an eye or a nose or a mouth. The signal is transmitted from cell to cell and may also shape the process as it unfolds: the voltage that means ‘eye’ might tell cells to start differentiating into a lens, a cornea, a retina, all while simultaneously shaping the eye’s overall organization. By revealing that specific voltages trigger specific organs, Adams’ video allowed Levin’s lab to read a few more grooves on the Rosetta stone of electrical signaling.
Levin’s team soon made use of this discovery. Against a wall of the room where Galvani’s drawings hang there are nearly a dozen rectangular Tupperware containers, stacked on metal shelving, each housing tadpoles of various ages. The tadpoles inside stretch to an inch long, their tails ending in transparent wisps that twitch to and fro. Buds that will eventually become legs push out of their abdomen. And, in one container, there are tadpoles that have a tiny round black stain where body melts into tail.
The tiny black dot, almost a perfectly round birthmark, is what Levin refers to as an ectopic eye — literally, one occurring outside of its normal place. This eye, the result of work published a year ago by Vaibhav Pai, one of the postdocs on the team, is growing on the tadpole’s abdomen. It functions, albeit not fully, and is connected to the brain by a thin highway of nerves. Pai prompted the eye to form there, and on other parts of the animal’s body, by changing the voltage on nearby cells to one that signifies ‘eye’.

These tiny, mutant tadpoles represent the first time anyone has succeeded in prompting a working eye to grow anywhere but the head, and researchers are already investigating whether these signals can be used to repair and regrow damaged eyes in mice. But for Levin, the path to regeneration in humans led next to limbs. His tadpole work had produced stunning results, but tadpoles can already regenerate tails; he was simply able to endow older tadpoles with the ability they’d lost. Would the same drug produce the identical result for frog’s legs, which don’t naturally regenerate, regardless of when they’re cut off? It was a slow process. Legs, unlike tails, can take half a year to regrow, and it took months of effort to determine the precise drug concentration they needed to drop into the water in which the frogs lived.
The results are just being published, in the January 2013 edition of the journal Communicative and Integrative Biology. In the article, a froglet’s hind section stretches across a photograph. Tiny digits — frog toes — push out from the skin, the final conclusive stage of frog limb regeneration. It was another significant increase in complexity, another step towards the goal of triggering regeneration in humans.
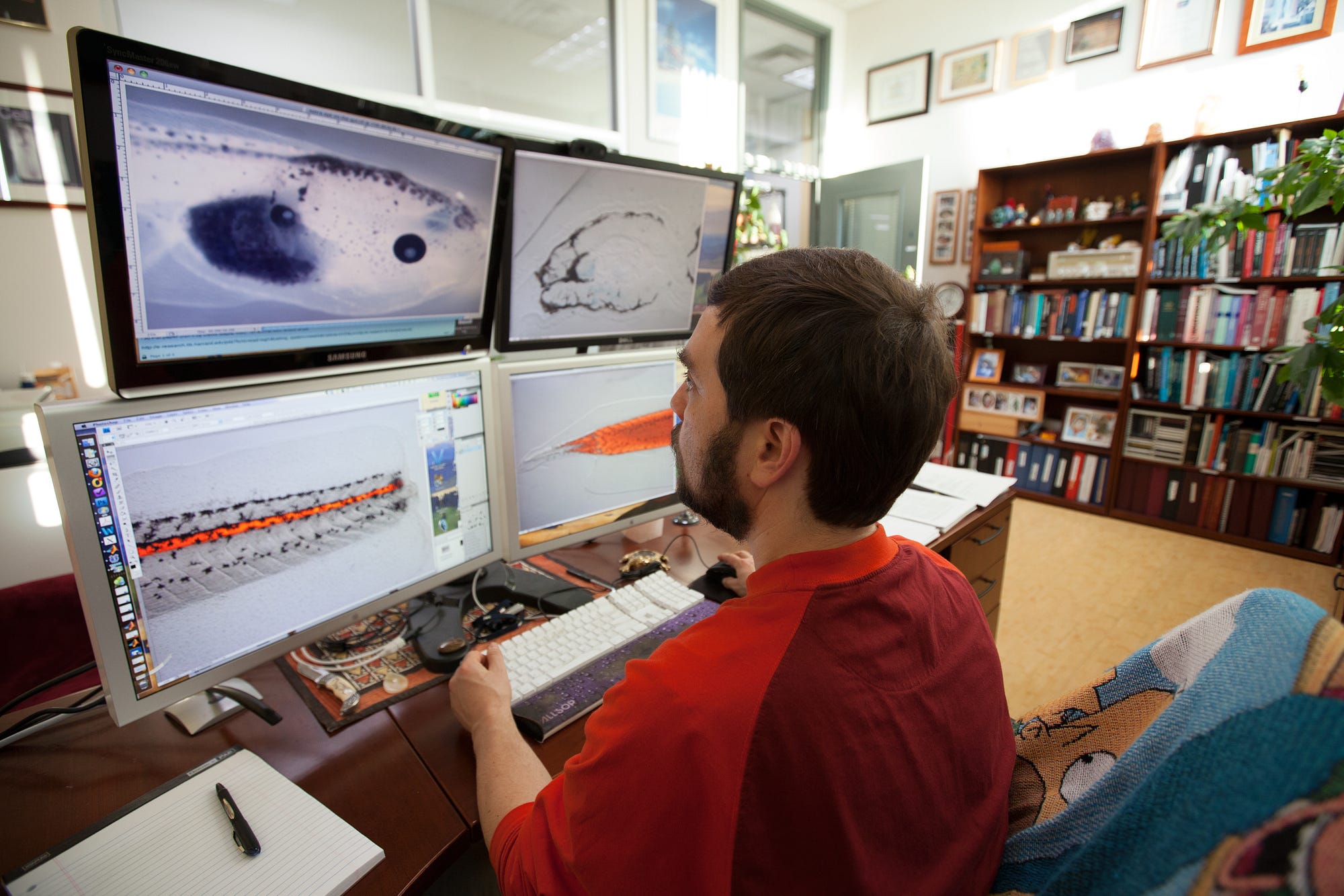
TODAY, LEVIN’S MOST CHERISHED MOMENTS come during his annual trips to the beach. Most years, he takes his parents to Florida in March, to a small resort town about halfway between Miami and Fort Lauderdale. It’s “a place for older people”, says Levin — meaning there’s none of the crazy student partying that is endemic at so many of South Florida’s beach communities.
These breaks are not really vacations; they’re time for what Levin terms “deep thinking.” “I used to bring a giant suitcase full of [printouts of scientific] papers,” he says — but these days, he’s able to pack it all on his iPad. Every day, Levin wakes at 5 am, has a quick breakfast, and then heads to the nearly deserted beach just as the sun begins to rise, where he hunkers down under an umbrella to “read papers and think and write down my ideas”. Then, at night, he explains his thoughts to his father over dinner. “If I can’t explain my ideas to a very smart non-expert then I haven’t really fleshed them out properly anyway,” he says.
It’s impossible to recreate this environment when he’s at home. Levin and his family live in Swampscott, a five-minute walk from where he grew up. Each day there are post-docs asking for guidance, lab equipment to check, grant applications to write. Levin does what he can, often waking at 4 am, when the streets are nearly silent and his two boys are asleep. During these precious minutes of quiet, he sits in a recliner in his bookshelf-lined office on the second floor of his home and just thinks. There are two monitors suspended on stands nearby, and when an idea occurs to him, he swings them into position above the chair, grabs a keyboard and takes notes.
These days, many of his early morning sessions are devoted to pondering the challenge of regeneration in mammals — which is where the biggest uncertainties about his line of research remain. Levin’s work is lauded by many, but a surprising number of regenerative medicine researchers remain unfamiliar with his efforts. When peers do voice skepticism, they tend to focus on the question of whether he’ll be able to replicate feats like leg regeneration in humans. “Mike’s work is fascinating, and potentially very important,” says David Mooney, a Harvard bioengineer. “How much of his data and approaches apply to mammals is an open question.”
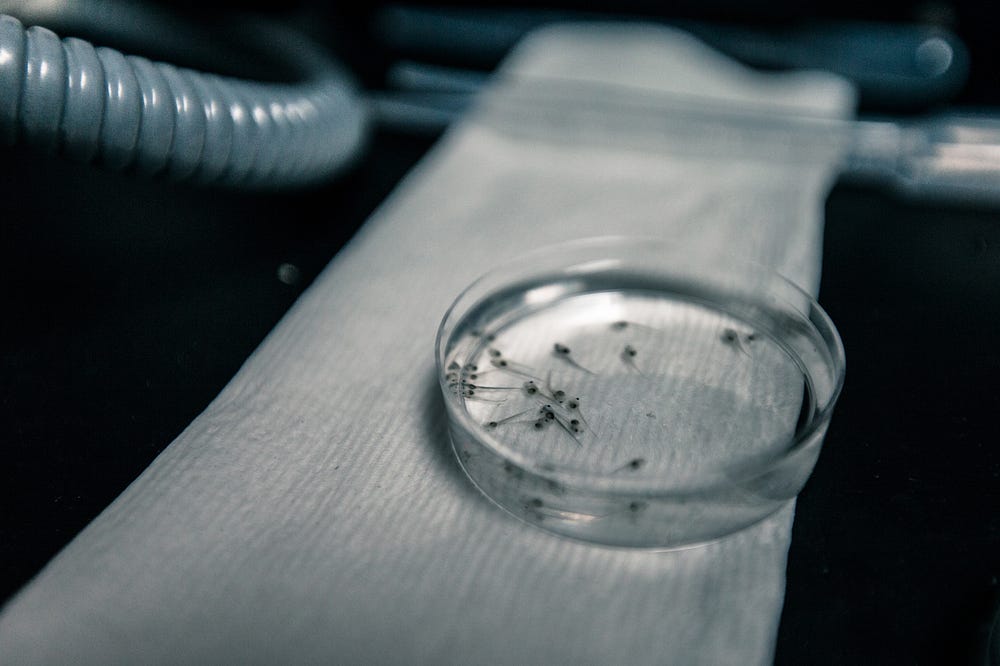
That’s why so much is riding on Levin’s next big research project. Sometime this coming spring, colleagues of his will remove fingers from some of the lab’s mice. Then they will apply drugs that, if all goes well, will trigger the regeneration of the missing digits. It’s a leap into the unknown and a huge test of Levin’s theories — perhaps the biggest so far. It’s also a study that could bring the possibility of human regeneration closer than it has ever been.
To get the drugs to where they are needed, Levin turned to David Kaplan, head of Tufts’s department of biomedical engineering, who designed a device that can fit over a mouse’s amputated finger without irritating it. The tiny cylinder, known as a BioDome, has a flexible plastic section that comes in three different sizes — mouse digits vary just as our own do. It is glued to a transparent tube that holds a few drops of liquid, just enough to bathe the stump in a fluid that includes drugs that alter cellular voltages. The BioDome is small and light enough that it can dangle from a mouse’s twig-thin finger without interfering as the animal scurries about its daily existence.
Whether Levin is able to achieve what was once considered impossible — prompting a mammal to regrow one of its digits — hinges on a slew of variables. Some involve the BioDome itself: how long can it withstand a mouse’s efforts to get it off? How long does it need to stay on? Others concern the cocktail of molecules that will wash over the animal’s amputated finger. Then there are the vagaries of animal development. It’s possible that regenerating a finger could be as simple as sending the right signal to the site of a wound. It’s also possible that scarring at the amputation site, or the mice’s inflammatory response, will prevent the limb from forming.
Levin and his colleagues know that they face significant hurdles — just as they know that, even if they do find success with mice, there’s no guarantee that they’ll be able to replicate the feat in humans. But when I ask Levin about this, he insists that he’s undaunted. “I read tons of science fiction as a kid,” he says. “But I think the reality is, I don’t know too many works of science fiction that are as wild and out there as things that have been discovered by real science.”
He pauses for a moment and then goes on. “The people who really think that it’s never going to work in humans don’t have a good grasp of how closely related we all are. Yes, there are differences, there are important distinctions. But the main lesson from biology is that the main stuff is all very similar. And there really is no principled reason to think it’s not going to work.”
We’re talking in his sun-drenched office. One wall is taken up by rows of bookshelves stacked with tomes about development, biology and bioelectricity. Photos of his family are framed in collages. Above his head loom four monitors, large enough for him to view images and data simultaneously. Plants frame the room with green. And, on the inside of the door, large bubble letters form a single word: “Believe.”
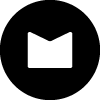
Originally published in December 2012 as “Electric Shock,” this story was written by Cynthia Graber, edited by Seth Mnookin, fact-checked by Sophia Li, and copy-edited by Lucy Odling Smee. Cynthia Graber narrated the audio version, with help from Scott Cawley. All photographs were taken by Kathi Bahr.
Follow Matter on Twitter | Like us on Facebook | Subscribe to our newsletter